Filtration membrane refers to a medium that separates two different phases of substances under certain driving forces. Under action of driving force, ions, molecules and some particles in the mixed solution can be separated by the permeability of the membrane.
According to the material type, membranes are classified into organic membranes and inorganic membranes. Organic membranes were first introduced and widely used in water treatment due to their high separation efficiency, simple equipment, and easy forming process. However, they still have inherent drawbacks such as short lifespan, poor chemical stability, weak resistance to microorganisms, and poor thermal stability.
Inorganic membranes, particularly ceramic membranes, have gradually attracted attention. Since the 1990s, the annual growth rate of inorganic membranes has reached 30%-35%, with ceramic membranes accounting for approximately 80% of this growth.
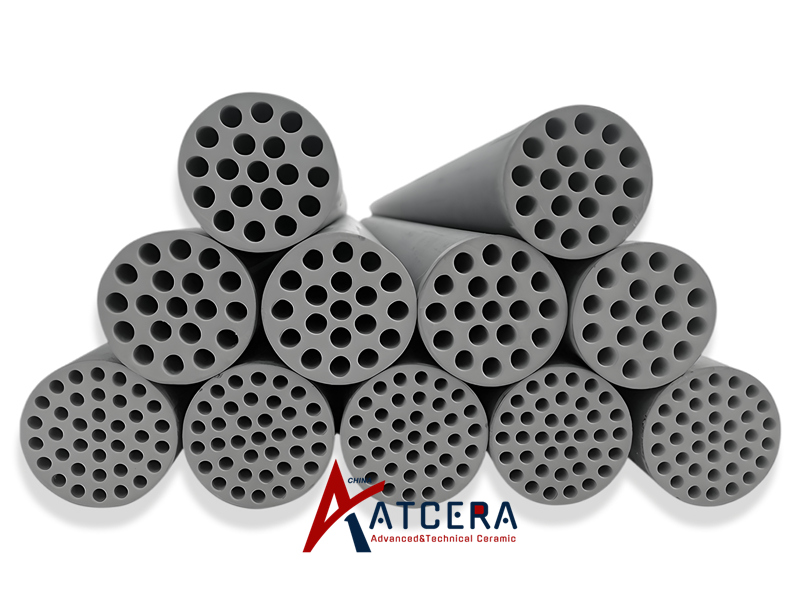
Performance Characteristics of Inorganic Ceramic Membranes
Ceramic membranes are a kind of solid-state membrane, with their support consisting of porous ceramic materials and the filtering layer composed of microporous ceramic membranes. They can be tubular, flat, or multi-channel shaped. The surface of ceramic membranes features uniformly arranged micropores with pore sizes typically ranging from 0.004 to 15μm. Prepared from materials such as Al2O3, TiO2, ZrO2, and SiO2, ceramic membranes are well known for their excellent chemical stability, high temperature resistance, and long lifespan.
1. High Temperature Resistance
Inorganic ceramic membranes have excellent heat resistance, with most ceramic membranes capable of operating at temperatures ranging from 1000-1300℃. They are suitable for the separation of high-temperature and high-viscosity fluids. In scenarios those chemical cleaning is not feasible, such as in the food, dairy, and pharmaceutical industries, or when need to reduce material viscosity through increasing temperature,
2. Good Chemical Stability
Inorganic ceramic membranes are resistant to acid, alkali, and biological corrosion, outperforming metal and other organic membrane materials in terms of corrosion resistance. They can be used to handle materials with extreme pH values, particularly alkaline materials, also have excellent antibacterial properties and resistance to biodegradation.
3. High Permeation Selectivity
Due to the small pore sizes of porous ceramic membranes, they have high permeation selectivity, and can be applied for ultrafiltration and microfiltration. Additionally, ceramic nanofiltration membranes have different separation characteristics for ions, so ceramic material could be selected according to the targeted ions to be separated.
4. No contamination, easy to clean, long service life
Ceramic membranes have excellent chemical stability, without phase changes or chemical reaction during the separation process, so there is no contamination to the liquid.
Ceramic membrane cleaning is quite easy, acidic, alkaline and enzymatic cleaners can be used to remove insoluble precipitates, oily substances and proteins on the membrane surface, steam and boiling water can used for sterilization, and backwash method can be applied to remove contaminants from the membrane pores, since ceramic membranes have asymmetric structure.
Ceramic membranes have excellent durability, typical lifespan is 3-5 years, and some can even reach up to 8-10 years with appropriate maintenance.
5. Photocatalysis
Certain types of ceramic membranes, such as TiO2 membranes, possess strong photocatalytic properties. Under ultraviolet light, they can kill bacteria and other microorganisms in the feed material, making them suitable for water treatment, air purification, and sterilization applications.
Application of Ceramic Membranes in Wastewater Treatment
1. Food Industry Wastewater
Ceramic membranes have high-temperature resistance, chemical stability, and resistance to acid, alkali, and biological corrosion, therefore they have a wide range of applications in food industry wastewater filtration treatment, mainly for manufacture factories of fruit juices, beer, soy sauce, vinegar, ginkgo water, tea, etc., also could be used for filtration of herbal medicines.
2. Textile and Paper-making Wastewater
Textile and paper-making wastewater is characterized by its large volume, high coloration, and complex composition, containing dyes, pulp, fiber impurities, heavy metals, and other substances, it has high biological toxicity and will cause severe pollution. Ceramic membranes is particularly effective to treat insoluble dyes, and for soluble dyes, the removal rate can be significantly improved by adding surfactant.
3. Biochemical Wastewater
Application of ceramic membranes in the field of biochemical engineering has recently attracted much attention, involving areas of cell removal, sterile water production, clarification of low-molecular-weight organic compounds, and bioreactor membranes. The use of ceramic membranes to separate bacteria from fermentation broth has resulted in multiple industrial-scale installations, only improving product productivity, reduces equipment load, but also significantly reducing wastewater discharge.
4. Oil-Containing Wastewater
Oil-containing wastewater originates from various sources, including petrochemicals, oil extraction, transportation, machining, leather, textiles, food, and pharmaceuticals. It is highly chemically oxygen-demanding and contains large amounts of oil, causing severe environmental pollution. Effective separation of oil-containing wastewater is crucial for environmental remediation, oil recovery, and water reuse. Ceramic membrane filtration technology has become a research hotspot due to its excellent separation effect and no secondary pollution.
5. Domestic and Urban Wastewater
Alumina membranes can be used to treat domestic and urban wastewater, micropores are not easy to be blocked, easy to clean, the trapped pollutants just stay on the surface of the control layer. After cleaning, the retention ratio and available flow rate can be nearly 100% recovered. Alumina membrane tubes with a control layer pore size ranging from 0.1-0.35μm have a removal rate of 83% for BOD5, 67% for CODcr, and 100% for solid suspended matter larger than 0.1μm.
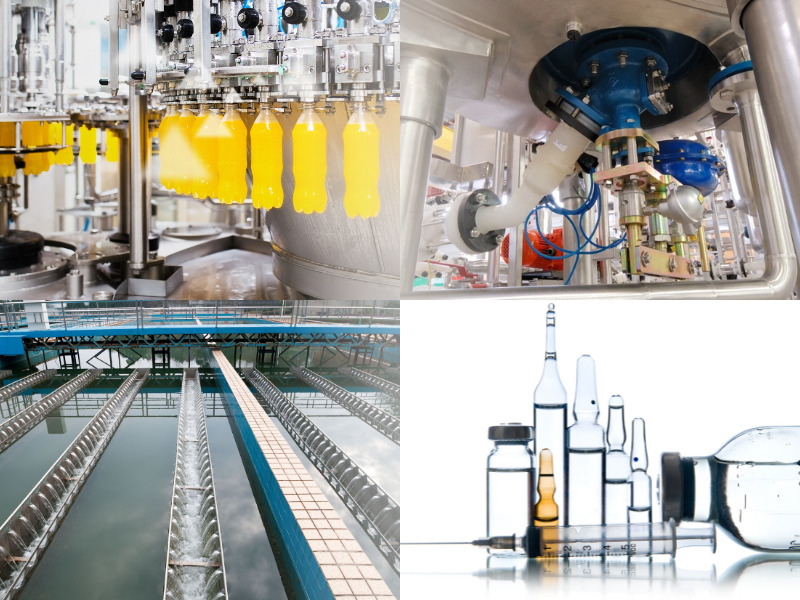
Application of Ceramic Membrane Bioreactors in Water Treatment
Application of ceramic membranes in water treatment is particularly widespread in the treatment of wastewater, mainly industrial wastewater, research and application of ceramic membranes in domestic sewage treatment are still relatively rare. The primary applications of ceramic membranes in domestic sewage treatment can be categorized into two types: membrane separation and ceramic membrane bioreactors (CMBR) that combine membrane separation with bioreactor technology. Currently, membrane separation is less commonly seen in applied research, with the majority of efforts focusing on ceramic membrane bioreactors, predominantly anaerobic processes.
Membrane bioreactor (MBR) is an innovative water treatment technology that combines membrane separation units with biological treatment units. Secondary sedimentation tank that used in traditional activated sludge method is replaced by membrane module, which is of high efficiency separation, unprecedented mud-water separation and sludge concentration effects are achieved. Additionally, the membrane efficiently retains suspended solids, organic matter, pathogens, and viruses from the wastewater, significantly improving the quality of the treated water.
Compared to traditional biochemical treatment techniques, MBR has significant advantages of high treatment efficiency, excellent water quality, compact equipment, small floor space, easy automation, and simplified operation and management. Since the 1980s, this technology has attracted increasing attention and become a hot topic in water treatment research. Currently, membrane bioreactors have been applied in more than ten countries, including the United States, Germany, France, Japan, and Egypt.
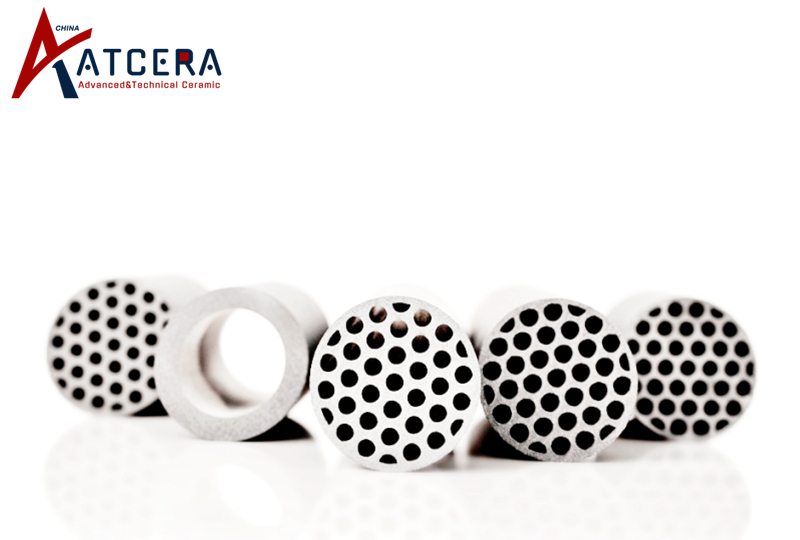
For centralized municipal wastewater treatment, the large volume of water to be treated and relatively low concentration of pollutants make membrane filtration be energy-intensive and economically less feasible. However, ceramic membrane bioreactors can be used for distributed treatment of domestic sewage, such as wastewater reuse in a residential complex. Ceramic membrane bioreactors offer stable operation, minimal maintenance requirements, high mechanical strength, long service life, and resistance to chemical corrosion, making them highly competitive compared to organic membranes.
In summary, ceramic membrane bioreactors have gained widespread praise in industrial water treatment due to their long service life, high reliability, consistent treatment effect, less operational and maintenance requirements, and have great application prospects in distributed domestic sewage treatment.